Their latest results have been published in the Astrophysical Journal.
Since the discovery of the first exoplanet in 1992, over 3600 exoplanets have been detected, yet the processes leading to their formation are not fully understood. It is widely accepted that planet formation occurs in protoplanetary disks composed of gas, dust and ice. Dust aggregation is a key step in planet formation but dust on its own is not very sticky. Models and observations suggest that water can act as a “glue" in planet-making processes.
Pressure-temperature gradients in protoplanetary disks mean that ices are continually processed, undergoing phase changes between different solid phases (amorphous, crystalline, mixture of both) and the gas phase. Previous studies have suggested that the structural properties of these icy particles could dictate collisional outcomes between protoplanetary disk particles.
The team of astrochemists employed neutron scattering at ISIS in combination with cryo-SEM (scanning electron microscopy) to characterise ice particle analogues used in laboratory collision experiments, and investigate whether changes in the bulk ice phase and/or surface structure correlate with the temperature dependencies observed in collisional data.
In preparation for the neutron scattering experiments, the team produced the icy-particles by spraying liquid D2O into liquid nitrogen, accumulating sample material for at least one hour. The particles were funnelled into a pre-cooled sample container, mounted in the neutron beam, and passively cooled at a constant pressure of 30 mbar. Samples were analysed on the Near and InterMediate Range Order Diffractometer, NIMROD.
Samples with two different mean particle radii were studied in experiments spanning the temperature range 103 K to 247 K. These conditions match the conditions under which collisional studies are performed in order to understand a variety of environments including protoplanetary disks, cometary surfaces and planetary rings. By exploiting the unique capabilities of NIMROD, the team were able to simultaneously characterise the bulk and surface structure of the micrometer-sized icy particles, and investigate structural changes with temperature.
“NIMROD, with its fast acquisition times and wide Q range, is able to provide structural information on a broad length scale", says Dr Helen Fraser, group leader. “This allows us to simultaneously characterise the particle ice-phase distributions on inter-molecular scales, their nanometer-scale surface properties, and their size and shape."
Scattering data reveal that the particles are mostly composed of hexagonal and cubic crystalline ice phases with small amounts of disordered inter-domain material. Changes in the distribution of these three distinct phases were observed as the samples were heated from 103 to 247 K. However none of the phase-change temperatures correlated with the collision temperature dependencies, and thus it was concluded that the crystalline bulk ice-phase does not influence collisional outcomes. Scattering data also revealed that the ice particle surfaces were not rough, as might be expected from the freezing process, and instead diffuse surfaces were observed. The thickness of the diffuse surface layer grew from 10 to 30 Å at temperatures above 210 K (Figure 1), meaning that the outermost water molecules had become more mobile and disordered than the bulk material. This phenomenon is well known in hail, ice and snow physics, where it is referred to as “surface pre-melting", but has received very little attention in planetary science so far. In addition to bulk ice-phase changes and surface pre-melting, sublimation and sintering of the icy particles were observed with increasing temperatures (Figure 1).
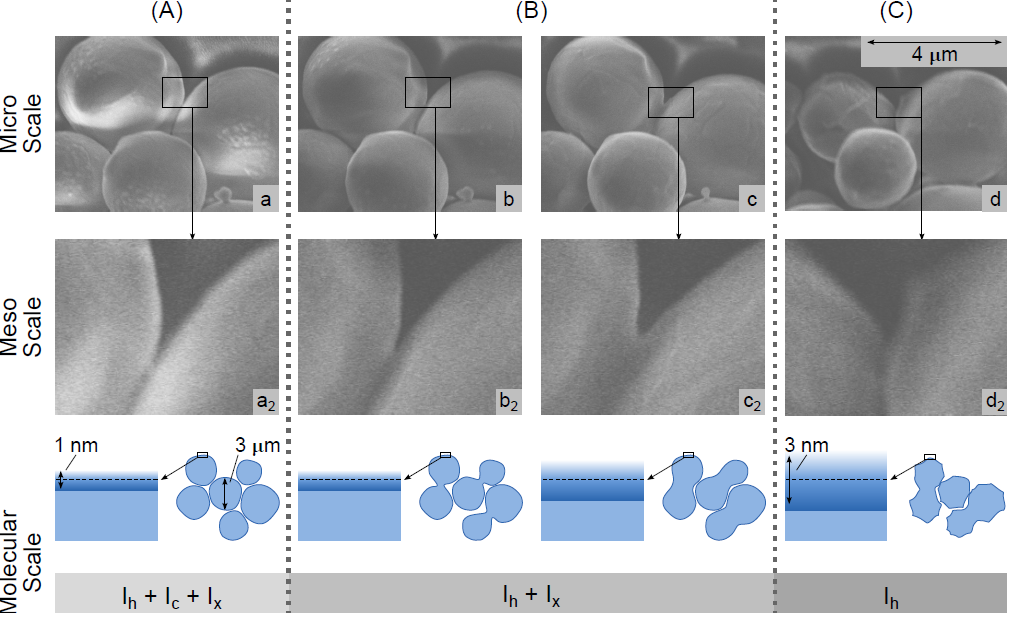 |
Figure 1. Schematic showing temperature induced changes observed in neutron scattering data from the icy particles in three distinct ice-phase regimes (where ih is hexagonal crystalline ice,ic is cubic ice, and ix represents inter-domain amorphous structures). Data are combined with cryo-SEM images. |
The observed collisional temperature dependencies suggest that the angstrom-scale surface properties of icy particles are affecting collisional experiments. Below 210 K collisional outcomes are temperature independent. However, beyond this temperature, the onset of temperature-dependent collision outcomes is observed alongside an increase in the thickness of the diffuse surface layer.
The results of this study have important implications for planet formation. Planet formation models currently prioritize parameters such as particle size and velocity but the results from this study suggest that the surface pre-melting process plays a key role in collision experiments at these temperatures. Consequently, the pressure-temperature environment may have a greater effect on collision outcomes than previously thought. Further investigations into the diffuse surface layer are required so that the influence of ice physics on collisional outcomes may be incorporated into more sophisticated models of planet formation.
“The experiments have suggested that the ice surface structure is fundamental to whether icy particles stick or not," says Dr Sabrina Gärtner, lead author. “The good news with what we have learned is that ice could act as a glue in planet building processes, but the tough news is that whilst the conditions most suited to ice-sticking are easy to obtain in the laboratory, they are unlikely to be found in space. In solving one mystery we have opened the door to many more questions and it's still not clear exactly how planets form."
.jpg)
|
Above Sabrina Gärtner on the NIMROD diffractometer.
|
Further information
The research paper published in the Astrophysical Journal can be found here.
Click here for an animation (narrated by David Mitchell) about how OU scientists are studying how planets form.