Porous materials such as Metal-Organic Frameworks (MOFs) have the potential to be used across a range of applications and industries for their ability to selectively adsorb and store gas molecules. For more information on porous materials and their uses, read our feature article.
By changing the chemical structure of these materials, it is possible to control their properties, leading to the preferential adsorption of particular gas molecules. A research collaboration, led by Professor Martin Schröder and Professor Sihai Yang from the University of Manchester, regularly uses neutron scattering experiments to understand how these changes in materials structure impact their gas selectivity.
In five recent studies in 2022, the group looked at systems that can capture and store ammonia. Ammonia is produced on a vast scale for agriculture and chemical industries, and also has the potential to be used as a carrier for hydrogen to support a carbon-zero economy. However, ammonia is highly toxic, reactive and corrosive, and therefore the storage material has to be very stable and inert.
MOFs could offer a solution to this challenge, with researchers working hard to design a highly stable MOF with properties that make it suited for reversible ammonia storage. A key part of the design process is understanding at the atomic level how ammonia binds inside the pores and, therefore, how this could be improved both to increase capacity and reversibility.
The group studied two systems using neutron diffraction experiments on WISH and inelastic neutron scattering on TOSCA, as well as other techniques including synchrotron studies at Diamond Light Source. Both systems show good ammonia adsorption, and the researchers wanted to find out why this was the case.
One study, published in the Journal of the American Chemical Society, focussed on the UiO-66 system. They studied the adsorption of the original system with a missing carboxylate ligand, 'UiO-66-defect', and with copper ions of different charges present: UiO-66-CuI, and UiO-66-CuII. They found that addition of the Cu(II) sites increased the adsorption of ammonia dramatically. Using neutron studies, they were able to 'see' that this was due to a strong Cu(II)···NH3 interaction. This is the first structural evidence of such an interaction. This discovery reveals the potential of using metal ions to target specific active sites for ammonia storage within these porous frameworks.
Graphic showing the different binding method of ammonia when the copper ion is present.
Another promising ammonia storage system, MFM-300(Sc), was also studied using neutron techniques. This material shows excellent reversibility over 90 adsorption/desorption cycles without degradation. Their structural and dynamics studies, published in Chemical Communications, confirmed that the material binds ammonia through interaction with -OH groups in the pore structure. Understanding that the -OH group is critical to the reversibility informs the design of future storage materials. This work was also selected as part of the Chemical Communications Pioneering Investigators collection.
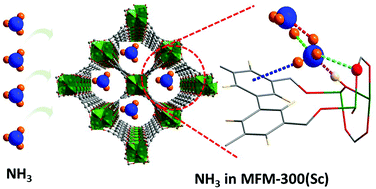
Graphic showing the binding of ammonia inside the pores of MFM-300(Sc)
As well as capturing gases when they are produced, MOFs can also be used to capture unwanted gases from mixed streams. One example is sulphur dioxide, SO2, which is produced during the combustion of fossil fuels. An advantage of using a MOF to capture SO2 in this process is that the adsorption is reversible, unlike current methods that produce large quantities of solid waste and waste water. This will enable the use of SO2 as a chemical feedstock.
In their study, published in Journal of the American Chemical Society, researchers from the same group carried out a comprehensive investigation of a series of Cu(II)-carboxylate-based MOFs with different pore sizes and various functional groups. Two of the MOFs studied, MFM-101 and MFM-190(F), showed very high SO2 adsorption, which was reversible over multiple cycles. Using neutron studies to compare the high-performing MFM-190(F) with MFM-126, which did not adsorb SO2 as well, they were able to see that the open Cu(II) sites present in MFM-190(F) but not in MFM-126 were the strongest adsorption sites for SO2. This explains the differences in their adsorption performance. The group was also able to use in situ synchrotron infrared micro-spectroscopy at Diamond Light Source to confirm how the gas molecule binds to the chemical species present inside the pores.
Understanding the way small molecules adsorb into these porous framework materials is also useful for the petrochemical industry. Separating the different components of an oil mixture after cracking is a crucial and highly energy-intensive process, with global ethylene and propene production currently around 200 million tonnes each year. Current systems use high pressures and very low temperatures, which require a lot of energy. A more energy-efficient alternative would be to use porous materials as adsorbents, selectively capturing one component over the rest of the mixture.
This selective adsorption was the focus of another study by the research collaboration, published in Chemistry of Materials. By adding a different transition metal ion into MFM-300 to create MFM‑300(In), they were able to selectively adsorb ethane from an ethane/ethylene mixture, which is a highly unusual observation. Again, the group collaborated with ISIS scientists to use the combination of neutron diffraction experiments on WISH and inelastic neutron scattering on TOSCA. They were able to see that the ethane molecules bind to the MOF differently to the ethylene molecules and bind to different positions in the pores. This configuration, combined with a larger pore size, enables the ethane molecules to interact more strongly with one another, improving the adsorption.
Through these studies, and their other work using neutron and X-ray scattering, the Manchester group have been able to make significant progress in the understanding of how pore geometry and chemistry links to gas adsorption. By understanding what influences selectivity and reversibility, their work will inform the design of future materials that will act as energy-efficient gas separators.
In addition to gas storage, these porous frameworks have applications in other areas relevant to industry and wider society. One example is proton conductivity, which is of relevance to proton-exchange fuel cell technologies. By confining sulphuric acid within the MOF, the conductivity is improved due to its strong acidity and multiple hydrogen donor/acceptor sites, which promote the formation of hydrogen-bonded networks.
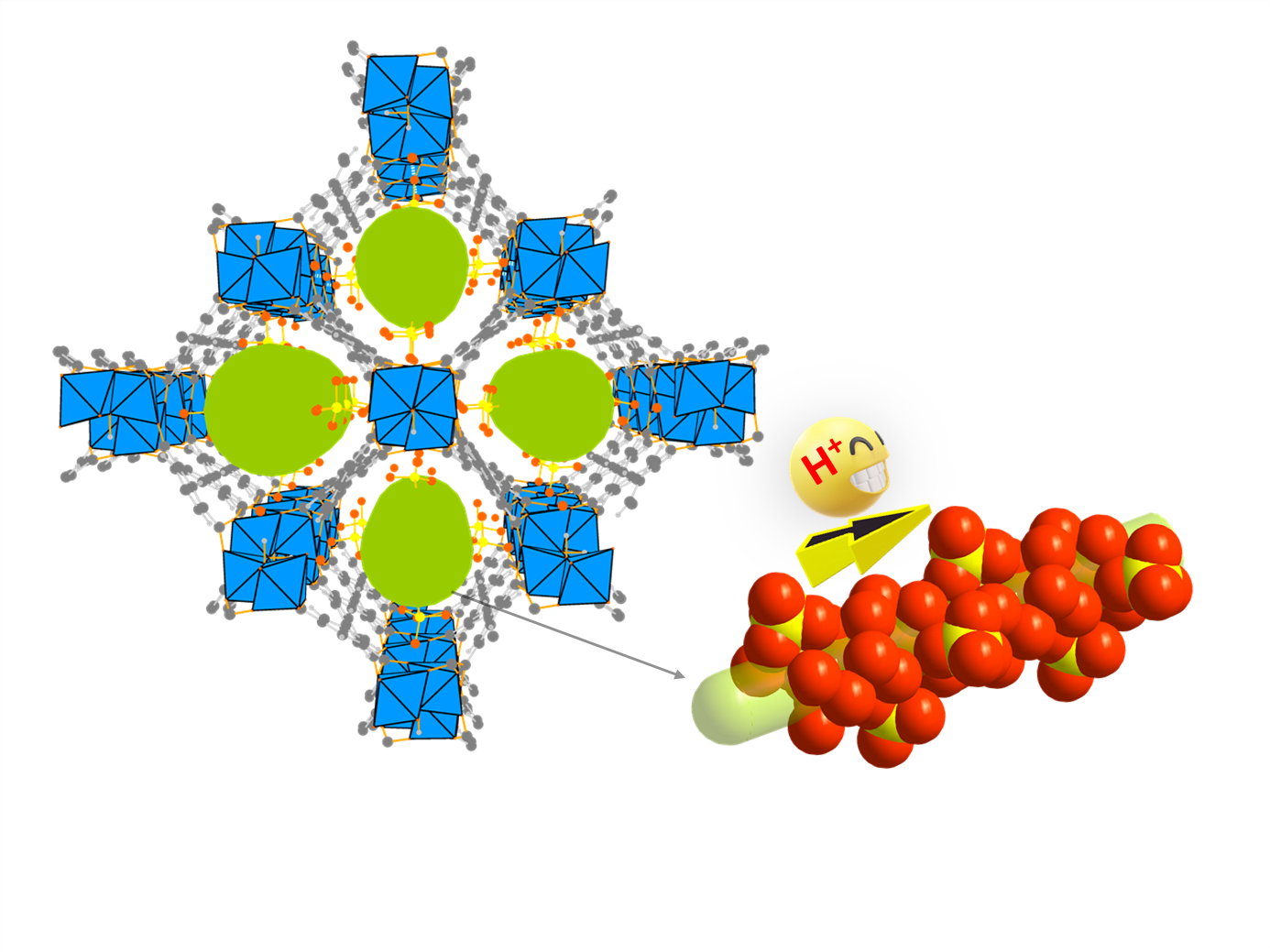
The Manchester group focused on MFM-300(Cr), adding sulphuric acid to create MFM-300(Cr)-SO4(H3O)2, which they then studied using high resolution synchrotron X-ray powder diffraction at Diamond Light Source and neutron powder diffraction on the WISH beamline at ISIS to characterise its structure. They also measured the conductivity using impedance spectroscopy, and then investigated the conduction mechanism in terms of the diffusion of protons using the IRIS instrument at ISIS.
Their experiments, published in Journal of the American Chemical Society, show that the MOF has a high proton conductivity. Combining their experimental results with computational modelling, they confirmed that this is due to protons moving within a hydrogen-bonded network created by the sulphuric acid and water molecules within the channels of the MOF structure.
Martin Schröder said "The studies enabled by scientists at ISIS and the Diamond Light Source have been essential in establishing and defining a real understanding of how our new materials function. Interdisciplinary and collaborative research is essential in driving real understanding of structure-property-function relationships".
Sihai Yang said “The development of advanced materials is of critical importance to our society and to the delivery of UK's 2050 Net Zero Target. Our research projects at ISIS and Diamond facilities have enabled direct visualisation of many complex, dynamic chemical processes at a molecular level, providing key knowledge to inform the design of new materials."

Further information
The full papers can be found online at:
DOI: 10.1021/jacs.2c00952
DOI: 10.1039/d2cc01197b
DOI: 10.1021/jacs.2c03280
DOI: 10.1021/acs.chemmater.2c01097
DOI: 10.1021/jacs.2c04900