To improve the materials used for such applications, it is important to understand the mechanisms behind ionic movement and how it can be improved. Methods of measuring ionic diffusion include nuclear magnetic resonance (NMR), electrical impedance spectroscopy and quasi-elastic neutron scattering. However, due to the way different methods probe different aspects of the ionic motion, the same material does not always give the same results across different techniques. Muon spin relaxation (μSR) spectroscopy is a powerful tool for studying ion migration in solids at the atomic scale, and has been used in many systems to probe diffusion mechanisms in solid-state materials for energy storage applications.
What is a muon and what can it do?
The positive muon, μ+, the heavier cousin of the positron, is far more commonly used in materials science studies and will be the focus of this article, although a recent study has combined the use of both positive and negative muons for measuring battery materials.
Muon spin relaxation (μSR) spectroscopy can be used to study various properties of materials using the interaction between the muon spin and their internal magnetic fields. Unlike neutron diffraction, where the neutrons pass through the sample and the change in their path is measured, muons are implanted into a sample. From their position deep below the surface, they are less susceptible to surface effects, enabling the investigation of the environment in the bulk of the material.
Muons are very sensitive to small magnetic fields in the areas in which they are implanted, giving crucial information about the microscopic properties of the material under investigation. This can be done not only for a sample prepared before an experiment (ex situ), but also during in operando investigations where the material is being tested during charge cycling. The photograph, above right, shows Innes McClelland preparing for the first in operando muon experiment at ISIS.
Because of their interaction with magnetic fields, muons can be used to study the dynamics of any ions that have a magnetic moment. These include lithium, sodium: common ions used in batteries, and hydrogen in fuel cell materials.
Battery Materials
Batteries are made up of one or more cells, each of which contains a positive electrode (anode), a negative electrode (cathode) and an electrolyte between them. When a device is turned on, a stream of positive ions flows across the cell from the anode to the cathode, with a flow of negative electrons flowing though the electric circuits. With a non-rechargeable battery, the chemical reactions involved only work in one direction; when the battery is flat, it's dead. Rechargeable batteries rely on different chemical reactions that can be reversed.
Measuring the movement of ions through a material, ion diffusion, is particularly important for battery materials, as this is a key indicator of the power and rate capability of a battery.
“One of the most important scientific problems to solve in our society is how to convert and store clean energy. In order to build better batteries, a breakthrough in science and technology is needed. Only recently, technical developments at large-scale experimental facilities such as ISIS have opened new possibilities for studying material properties in a straightforward manner."
Jun Sugiyama, Toyota Central Research & Development Laboratories, Japan and Dr. Martin Månsson, KTH Stockholm.
Lithium ion battery electrodes
The dimensionality of diffusion pathways in battery electrodes can have a significant impact on electrochemical performance. Different cathode materials have different crystal structures, which offer different routes to lithium diffusion through the material. Some examples are shown in the figure below.
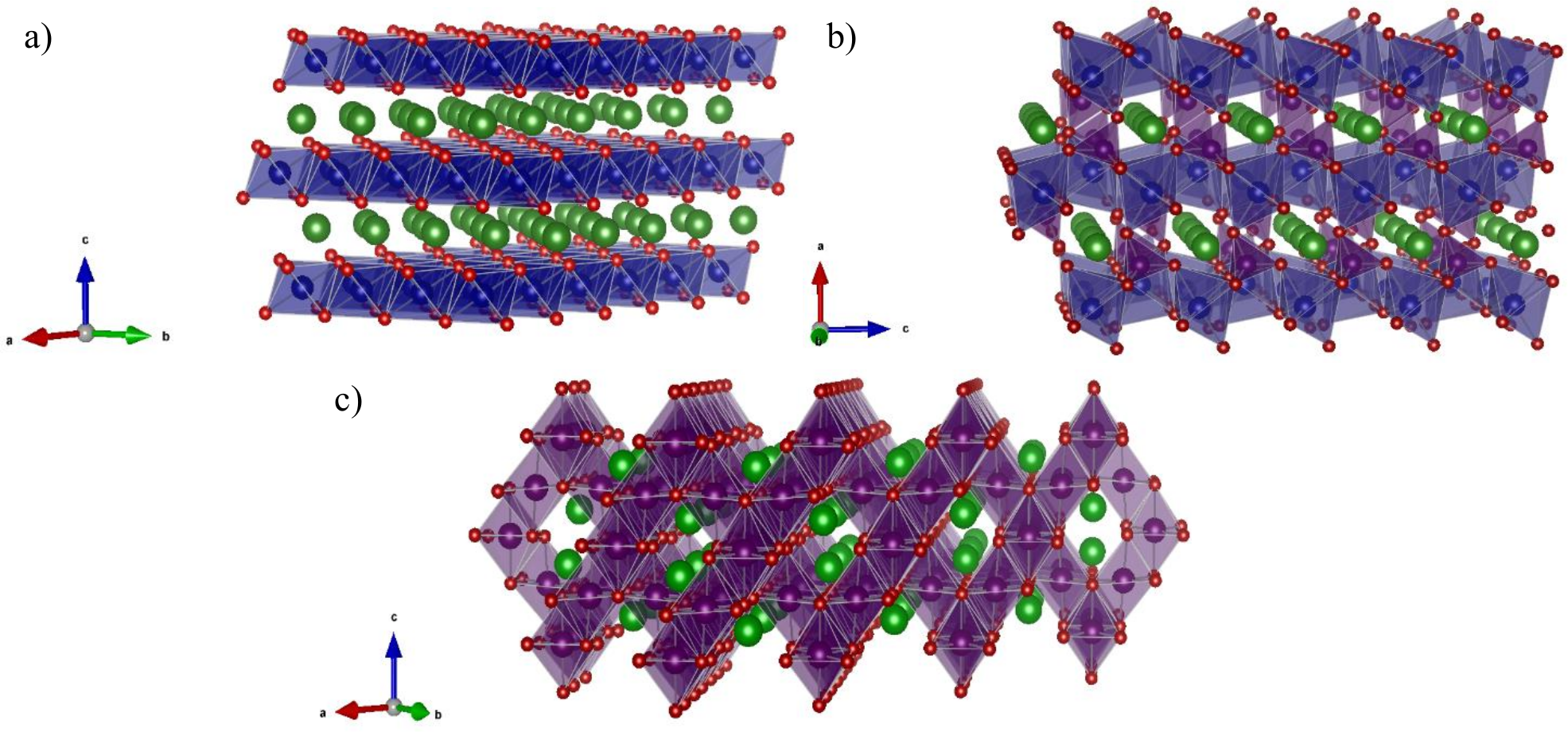
Structures of common cathode materials illustrating the diffusion pathways for Li+ ions (green spheres): (a) spinel framework LiMn2O4, where Mn and O are purple and orange spheres, respectively; (b) layered metal oxide LiCoO2, where Co and O are blue and orange, respectively; (c) olivine structured LiFePO4, where PO4 and FeO6 are brown tetrahedra and blue octahedra, respectively.
By making small changes to the composition of the cathode materials and then measuring the diffusion of the lithium ions through the structure, research groups have been able to identify areas where improvements can be made, and quantify the effect of any changes on the ability of a material to act as a cathode. ISIS muon scientists are part of the FutureCat project, which will coordinate cathode chemistry design, development and discovery.
Although not studied as extensively as cathode materials, anodes such as intercalated graphites have been studied recently using μSR to investigate the diffusion properties. The technique has also been used for studying possible alternatives to graphitic anodes such as doped transition metal nitrides, where other microscopic diffusion measurements struggle due to the interaction with the metal paramagnetic moments.
Electrolytes
There are current safety issues related to liquid electrolytes in lithium-ion batteries, leading to the search for alternatives including polymers, composites and solid-state electrolytes. If a suitable solid electrolyte was found, then the anode could be replaced by lithium metal. μSR experiments have been used to probe the possible diffusion pathways for lithium ions inside garnet materials, which could be used in the future as solid-state electrolytes.
Sodium ion batteries
Due to the relatively low price of sodium in comparison to lithium, sodium batteries could be a cheaper alternative if they can reach the same performance, although the heavier sodium ion means that the more likely application is for stationary energy storage.
Experiments using μSR have been carried out to investigate both adapted lithium-ion cathode materials and novel structures for sodium-ion cathodes, and to see how these structures could be changed to improve performance.
The future
The study of diffusion of hydrogen, lithium and sodium ions using μSR is now well established within the community, and there is the potential for this to be expanded to include other ionic species including magnesium, aluminium and iodide.
Muons implanted into a material offer a great way to investigate its bulk properties. However, with developments in producing thin films of the interfaces in battery materials, then low-energy muons that travel up to 200 nm into samples can be used to probe the ionic motion in these situations.
As muon facilities undergo technological improvements, the rate of muons delivered to the sample is increasing. This has enabled more detailed studies of ionic motion in areas that are well established, such as lithium-ion diffusion, and enabled the study of battery materials in operando during charging and discharging. Further improvements will allow commercially relevant charging and discharging rates to be accessed as well as making existing studies more precise.
As well as muons, neutrons are a crucial tool to investigating battery materials, as they give a view of how light elements such as lithium are moving around, and better resolution between some of the other elements that are commonly found in Li-ion batteries (such as iron, nickel, cobalt and magnesium). ISIS therefore plays a key role in developing battery materials for the future.
Further information
A recent review paper looks back at applications of positive muon spin relaxation (μSR) spectroscopy as an active probe of ion diffusion in energy storage materials.